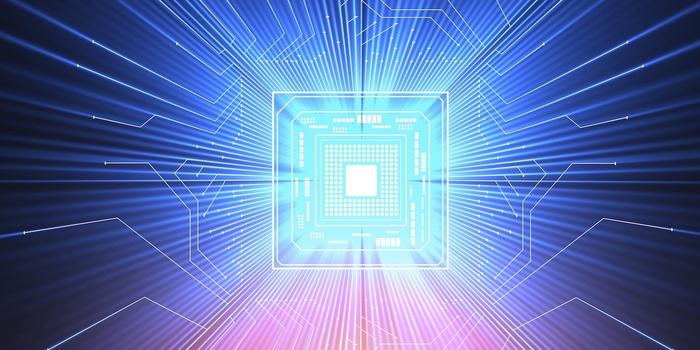
Technology has the power to transform our daily lives. In this three-part series in partnership with Intel®, we’ll bring you stories of the cutting-edge innovations that are doing just that—and the people working to make those innovations a reality. Check out Parts 1 and 2, and read on for Part 3.
Quantum computing can seem like cold fusion or hydrogen cars—the kind of wildly futuristic technology that always feels years away. But physicist Dr. Anne Matsuura is working to make it a reality. As the Director of Quantum Applications and Architecture at Intel Labs, Matsuura leads a team that is building out this long-promised advance in computational power—that has the power to change the world.
It’s hard to overstate the impact the next leap in computing could have. It could drive the discovery of new materials, accelerate drug discovery, and maybe, someday, help us predict natural disasters. Many experts believe they will find applications in financial markets, specifically to calculate options pricing and risk analysis by considering far more data than a typical computer could.
Some of these advancements are years away—but Matsuura’s work in building the system architecture of a true quantum computer is already laying the fundamental groundwork.
The Power of Quantum Computing
According to Matsuura, quantum computing will be most impactful when it comes to understanding systems and materials that have quantum characteristics themselves. “These materials are really hard or impossible to simulate classically,” Matsuura says. That’s because the interactions of the electrons within them require computational horsepower beyond the reach of even the biggest supercomputers of today.
Take superconductors, for example. These materials have the ability to conduct electricity with zero resistance. While there are some modern-day uses—superconducting magnets currently power MRI scanners and a number of futuristic high-speed trains in Asia—they are impractical because to work, most of them must be cooled to cryogenic temperatures near absolute zero (that’s minus 460 degrees F!). But while low temperature superconductors are well understood, but there are certain materials that are superconducting at higher temperatures that are not.
A quantum computer could not only simulate how higher-temperature superconductors work, but also predict entirely new materials that could have exotic properties, including the ability to be superconducting at room temperature.
Quantum computing can also be applied to developing new molecules and discoveries in the world of chemistry, including lifesaving drugs. “Pharmaceutical drugs could be an extension of chemistry,” Matsuura says. “If you can simulate complicated chemicals and materials, then maybe you can simulate new drugs.”
And renewable energy technologies could also become more affordable and efficient thanks to quantum computing, which could help identify new catalysts and simulate chemical reactions such as those needed to create solar fuels.
How Quantum Computing Works
Quantum computers get their superpowers from a few quirks of quantum mechanics. Whereas a bit that stores data in a typical computer can be either a zero or a one, a “qubit”—the way a quantum computer stores information—can exist in multiple states simultaneously. These qubits are paired up through a process called entanglement, which says that two different particles can be connected even when they’re physically separated.
Building a quantum computer is a major challenge of basic science and computer engineering. Qubits are fragile and fleeting and moving them around to perform computations can lead them to lose their “coherence,” which means they are no longer entangled. Therefore, it’s difficult to run quantum computing with more than a few dozen qubits—at least for right now.
“At Intel, we’re creating our algorithms so that they can scale up and you can use them on many, many more qubits when those become available,” Matsuura says.
The Right Team for the Job
A true quantum computer needs advanced technology to create qubits and entangle them, but it also requires the rest of the computer system, including the processor and the control electronics. And the project requires many kinds of expertise. “Multidisciplinary talent comes together at Intel Labs,” Matsuura says. “Some people on the team are physics researchers straight from academia and others are engineers who have built products before.”
Matsuura’s team partners closely with another team of quantum researchers in Intel’s Components Research Group that focuses on building the core qubit technologies and control systems for these devices. Together, they are eyeing an ambitious goal: quantum practicality, which is the point at which quantum computers can be scaled enough to have meaningful commercial applications.
Matsuura herself has a wide-ranging background. After working in academia, she became a strategic adviser who helped venture capital and other firms decide where to invest in advanced technology startups involved in nanotech, photonics, and sensors. Later, she served as the chief science officer for an optics-based nonprofit and as chief executive for a startup that offered support for understanding advanced materials science. When Intel asked her to come aboard to spearhead a quantum computing project, she was intrigued by the idea of working at a major technology company on something that still requires fundamental research.
Intel’s computing heritage provides a foundation for tackling the huge technological hurdles inherent in building a real quantum computer. Intel builds their qubits from silicon-based quantum dots, tiny nanometer-sized crystals that have quantum properties such as the ability to change energy states when illuminated by ultraviolet light. It’s a process that Matsuura says is remarkably similar to the one Intel used to build the silicon transistors that were created for the PC revolution (and are still produced today).
The Best Is Yet to Come
Though we may be a decade from seeing commercially viable quantum computers, Intel Labs has been reaching important advances to make the field a reality sooner rather than later.
One project, the Intel Quantum Simulator (IQS), is an open-source environment that can simulate the interactions of up to 40 qubits. Matsuura’s team uses IQS to develop quantum algorithms (or quantum programs) to run on qubits. This type of research enables Intel to understand the system architecture necessary for a useful quantum computer and has proven invaluable to building a real-world prototype “full stack” quantum computer testbed, which will contain all the components a viable quantum computer would require. Intel is scheduled to deliver such a full-stack prototype testbed, called Q-NEXT, as part of a partnership with the U.S. Department of Energy Quantum Information System Research Center toward the end of 2021.
These Intel innovations will be key to achieving the potentially world-changing applications of large-scale quantum computing. To Matsuura, one of the most exciting parts is that it’s not entirely clear what those applications are. The technology may do things no one has thought of yet, much like the very early days of traditional computers.
“It wasn’t clear [at the beginning] that the classical computer was going to be useful. There was a stage when it took up a full room,” she says. “That’s where we are now with quantum computers. We have ideas, but nobody really knows how we’re going to use these machines.”